Erythrogenic Effects of Testosterone
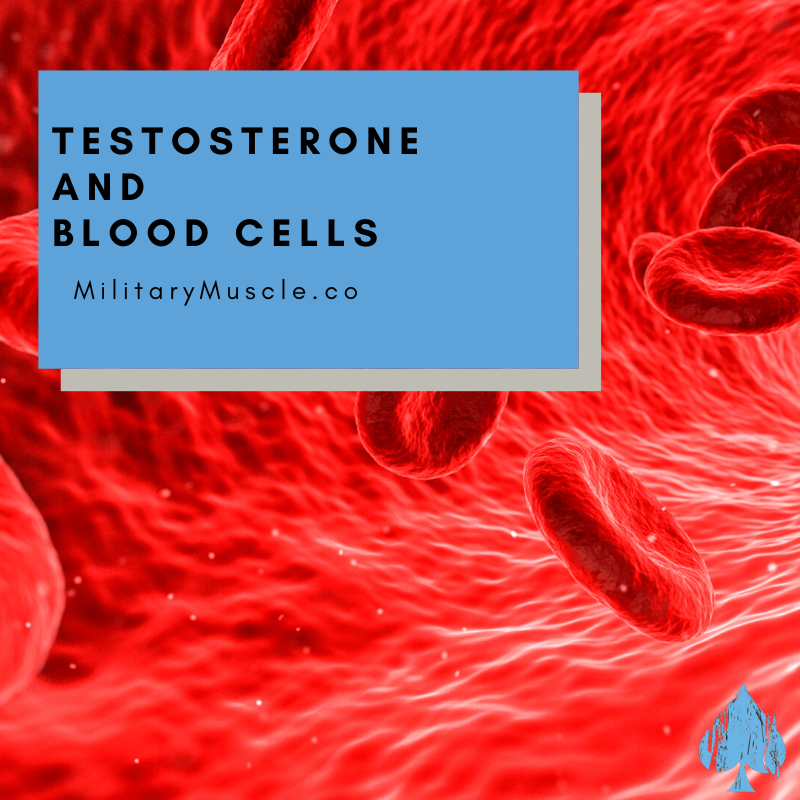
Written by Ben Bunting: BA, PGCert. (Sport & Exercise Nutrition) // British Army Physical Training Instructor // S&C Coach.
--
Testosterone can stimulate erythropoiesis in the bone marrow via direct effects on bone marrow stem cells, IGF-I induction, and androgen receptor-mediated mechanisms. These mechanisms work through the hormone erythropoietin. This hormone is produced by interstitial cells in the kidney and acts on red blood cells and bone marrow stem cells to promote erythropoiesis.
Erythrogenesis Explained
Erythrogenesis is the process of erythroid cell development. Erythroid progenitors can be classified according to their ability to form a characteristic colony-forming unit (CFU-GEMM). In addition, retinoic acid and dexamethasone are essential during erythropoiesis. Finally, GATA1 is an important regulator of erythroid lineage commitment and terminal differentiation.
Erythropoiesis is a complex multistep process involving a series of gene expressions and growth factors. It begins with erythroid uni-lineage commitment, and proceeds to proliferation and maturation of committed progenitor cells. This process requires a highly coordinated and tightly regulated system to ensure homeostasis and the proper production of hemoglobin molecules.
The human body produces about 2.5 billion red cells per kilogram of body weight per day. The process of erythropoiesis is tightly regulated and carries out at a constant rate to ensure adequate red blood cell production. However, erythropoiesis can go wrong. As a result, the production of red blood cells can be stunted.
Red blood cells are produced continuously in certain bones, called bone marrow. In the second gestational month, this process moves to the fetal liver. Once the baby is born, erythropoiesis continues in the bone marrow. During this stage, mature red blood cells express the adult forms of hemoglobin and deliver oxygen to tissues. These red blood cells circulate throughout the body for about 120 days, after which they are destroyed by macrophages.
Erythropoiesis can also take place outside of the bone marrow. In certain diseases and animals, it occurs in other parts of the body, such as the liver and spleen. This process is referred to as extramedullary erythropoiesis.
Erythropoiesis Explained
There have been many advances in our understanding of erythroid cells, especially as they undergo expansion and terminal differentiation. Much future research will further our understanding of the molecular control of erythropoiesis. The following is a collection of references that will aid in your understanding of this complex process.
b1 integrin
PIEZO1, a non-selective mechanosensitive ion channel, is implicated in erythropoiesis. Although it is not exclusively expressed in erythrocytes, it is expressed more highly in precursors of erythroid cells, indicating its potential role in the process. PIEZO1 is also involved in the activation of downstream Ca2+-mediators, including calpain and PKC.
During fetal development, a4b1 integrin is important for the expansion of erythroid progenitors. The same applies for the terminal erythroid differentiation of adult cells. Several studies have revealed a critical role for a4b1 in the development of erythroblasts and the regulation of basal erythropoiesis.
Necessity of a large number of erythroid progenitors
The generation of erythroid progenitors is a critical process in erythropoiesis. In vertebrates, there are two morphologically distinct lineages of erythroid cells. These cells are important for normal development, and have evolved to perform highly specialized functions such as transporting oxygen, defending against oxidation, and remodeling the blood vessels. This development is a key aspect of ontogeny in mammals.
This process begins with the formation of progenitor cells called erythroblasts. The next step is the differentiation of these progenitors into reticulocytes. The two distinct types of erythroid progenitors have been identified through colony assays, and have distinct functional characteristics. Proerythroblasts undergo three to four mitoses to mature into reticulocytes, and orthochromatic erythroblasts undergo reduced cell size and enhanced chromatin condensation.
Effect of decreased apoptosis on erythropoiesis
The regulation of erythropoiesis involves a balance between the production and destruction of erythroid cells. Recently, increasing evidence has been found to implicate apoptosis in this process. This regulation involves two signaling pathways: the mitochondrial dependent intrinsic pathway and the death receptor dependent extrinsic pathway.
When apoptosis occurs, the red blood cells in the bloodstream die. These dead cells are removed from circulation. The process of eryptosis is an example of a stress-induced programmed cell death. In eryptosis, the damaged cells are removed from circulation and undergo morphological changes, such as shrinkage and membrane blebbing.
Testosterone increases red blood cell count
Testosterone has been shown to increase red blood cell count and erythrogenic effect in various human studies. In one study, testosterone administration increased the number of red blood cells, platelets, and EPO in a group of young men. It also decreased the concentration of ferritin and hepcidin. These side effects were not observed in the placebo group.
Testosterone is also thought to affect erythropoiesis, or the production of red blood cells in the bone marrow. This happens via a number of mechanisms, including IGF-I induction and androgen receptor-mediated mechanisms. The process of erythropoiesis involves red blood cells and the hormone erythropoietin, which acts on them.
Testosterone has several other important roles in the body, including regulating the sex drive, bone mass, fat distribution, and muscle mass and strength. It also converts to estradiol, a form of estrogen. However, the excessive iron in hemochromatosis can reduce testosterone levels, resulting in hypogonism or hypogonis.
In addition to increasing red blood cell count, Testosterone may also increase hemoglobin levels. Regularly undergoing a complete blood count will help ensure that testosterone levels are in the proper range, and blood cell counts will remain normal. While this side effect is rare, it is important to note that high levels of red blood cells can make the blood thicker than it should be, which can have harmful effects.
There have been several studies examining testosterone's erythrogenic and hemostatic effects. One study by Hellem AJ, Borchgrevink CF, Ames SB, and Ames JS examined the role of red blood cells in hemostasis and the effect of blood viscosity and flow on the red blood cell count. The findings suggested that testosterone increases Hct and Hb by as much as three and a half times, respectively.
However, these studies have not explored the effects of testosterone on anemic participants. To determine if testosterone has different effects on anemic men, subgroup analyses were conducted on anemic and nonanemic men. Nonanemic men were those with hemoglobin levels of at least 13 g/dL. Anemia was defined using World Health Organization criteria. In nonanemic men, iron saturation was normal and the mean corpuscular volume was normal.
That said, while testosterone may have benefits for treating anemia, but it can also cause a number of dangerous side effects. For example, testosterone treatment (TRT) has been linked to an increased risk of blood clots. In fact, a recent study found that men who take testosterone have a 63 percent increased risk of developing blood clots in their veins. Luckily, there are a number of treatment options available to help reduce the risk of venous thromboembolism.
Testosterone increases action of platelets
Testosterone is a powerful hormone that can increase the action of platelets. Studies have shown that testosterone affects platelet function, coagulation, and fibrinolysis. One study found that testosterone increased platelet aggregation and inhibited fibrinolysis in adult rats. The rats were also found to have shorter bleeding, clotting, and prothrombin times.
Testosterone also increases the number of neutrophils and monocytes. This suggests that testosterone promotes differentiation of hematopoietic progenitors into the myeloid lineage. These results have potential therapeutic implications.
It has been suggested that testosterone increases the production of thromboxane A2 receptors on platelets. However, this mechanism is not entirely understood. The hormones are not the only factors influencing platelet reactivity. There are other vasoactive molecules that influence platelets, including estrogen.
In addition, testosterone increases the number of blood platelet cells in the body, making the blood more prone to clotting. This can lead to an increased risk of heart attack and stroke. Furthermore, testosterone is also known to affect the immune system and fat metabolism. Therefore, it is important to consider the risks associated with increasing your testosterone levels abnormally high when deciding on the best testosterone therapy for your condition.
A study also showed that testosterone can modulate the expression of COX1 and ERbeta in platelets from both men and women. While the effects of testosterone on blood platelet function are not clear yet, the findings are interesting. Furthermore, it is important to note that testosterone affects the expression of these proteins in megakaryocytes.
While this is a promising study, the study also revealed that testosterone administration increased both the monocyte and erythrocyte counts. In addition, it also increased the TXA2 receptor density in platelets. The increased TXA2 receptor density was positively correlated with pretreatment testosterone levels.
While it is not clear whether testosterone increases the risk of heart disease, research has shown that it doubles the risk of deep vein blood clot. This risk is present even in middle-aged men with no signs of hypogonadism. It is also more pronounced in seniors.
Testosterone increases iron utilization for erythropoiesis
Testosterone regulates erythropoiesis by stimulating the release of a protein called erythropoietin (EPO) from the kidney, which in turn stimulates bone marrow to make more red blood cells. Testosterone can also increase the level of a hormone called hepcidin, which controls iron absorption in the body. Because iron is an important component of red blood cells, a reduction in this hormone may lead to increased iron absorption.
Testosterone is also associated with an increase in hematocrit. The increased hematocrit levels associated with testosterone therapy are related to increased levels of EPO. Furthermore, testosterone increases the level of erythropoietin and hemoglobin, two markers of erythropoiesis.
Recent studies have shown that testosterone therapy improves erythropoiesis and iron utilization in hypogonadal men. However, the evidence is inconsistent. Most studies reported that testosterone increases EPO levels but did not increase iron levels, and many were conducted with small samples and variable treatment durations. Moreover, they have not measured serum EPO longitudinally. In one study, the serum EPO levels of men treated with testosterone increased but tended to fall back to baseline values at six months. However, serum EPO levels remained abnormally elevated in relation to elevated hemoglobin and hematocrit levels. Moreover, the studies also found that testosterone administration shifted a typical log-linear negative correlation between serum EPO levels and hematocrit levels.
The iron supply is essential for the production of hemoglobin. Without adequate amounts of iron in the blood, the iron-transferrin compartment depletes rapidly. Without an adequate supply of iron, hemoglobin synthesis and other iron-dependent processes are inhibited. This modulation of iron homeostasis is important for the body. However, this process is still poorly understood, and the exact mechanism involved is still unclear.
Hepcidin is the major circulating iron regulator. It controls major iron flows in the blood plasma and regulates the release of ferroportin. In contrast, erythroid precursors secrete mediators that suppress the production of hepcidin in the liver and increase the amount of iron available in the bone marrow. Although hepcidin is regulated by inflammation, its role in physiologically and pathologically stimulated erythropoiesis is unclear.
The iron is transported to the bone marrow by a protein called transferrin. The iron then joins with the protein ferritin, which is found in the spleen. This iron is used to make red blood cells. But, after 120 days, the red blood cells degrade in the spleen and the iron is then recycled.
The transferrin compartment of the red blood cell contains about three milligrams of iron at any given time. This compartment turns over every couple of hours. In humans, most iron required for erythropoiesis comes from the recycling of senescent red blood cells by macrophages. This process provides 20 to 25 mg of iron daily. The rest of the iron consumed is absorbed by the intestinal tissues, which amounts to approximately 1 to two mg, or 0.05% of total body iron. Although this is not a large amount, it is enough to compensate for the loss of iron.
Conclusion
Testosterone has the potential to increase the red blood cell count in humans. It increases the production of erythropoietin, which tells bone marrow to make more red blood cells. In addition, it may influence the metabolism of iron, which is important in red blood cell production.
A patient's hematocrit (red blood cell count) should be checked regularly and should be measured after beginning testosterone replacement therapy. If the hematocrit is higher than 50%, the dosage of testosterone should be reduced. Patients should also be monitored for symptoms of polycythemia, such as ruddy skin and easy bruising. Some patients may also experience epistaxis.
One of the most important findings of this study was that testosterone increases the red blood cell count in both sexes. Testosterone treatment increased the EPD in male mice and female mice, and increased the hematocrit in female mice. Other RBC indices were increased as well. Testosterone treatment also increased whole-blood viscosity. A maximum increase of approximately 13% was noted at 15 s-1 systolic shear rate. The difference between the groups was not statistically significant. However, testosterone treatment increased erythroid deformability in female mice.
Testosterone treatment can increase hemoglobin levels, which helps prevent anemia. In addition, studies have shown that the risk of mortality from anemia is almost doubled if the condition is mild.